The origin of oxygen
There’s a bit of a persistent rumour getting around on internet and extranet circles. Actually far too many, but today’s lucky topic is the notion that plants produce oxygen from carbon dioxide.
It’s a simple enough story. We know that photosynthesis consumes carbon dioxide, and produces surplus oxygen. Since CO₂ quite obviously contains O₂, well, there’s not even anything to question.
Except that photosynthesis has a few more aspects than just these two gases, and the whole system isn’t an atomic process.[1] Fortunately you don’t need to be within arm’s reach of a Biochemical Pathways poster to grasp the overview.
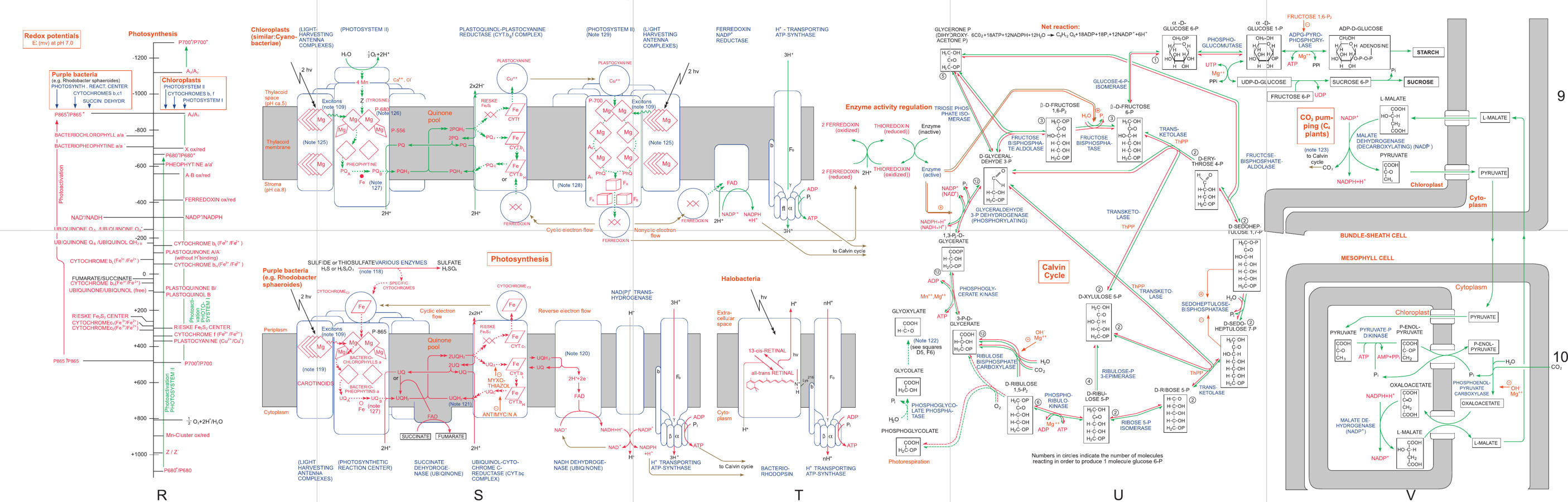
Photosynthesis, clear as chlorophyll!
This diagram erroneously labels two complexes as ‘Photosystem II’, when the one on the right involving P-700 and ferredoxin should be labelled ‘Photosystem I’. From Roche Biochemical Pathways, 4th Edition, Part 2: Cellular and Molecular Processes.
There are two broad aspects to photosynthesis, helpfully classified as ‘light-dependent reactions’ and ‘light-independent reactions’.
The function of the light-dependent reactions is to use energy from light to synthesise ATP from ADP and inorganic phosphate, and in many organisms, to reduce NADP⁺ to NADPH as well. The meanings of these acronyms isn’t too important, but what does matter is that ATP and NADPH are used by pretty much every metabolic process as a sort of chemical currency, being left as ADP and NADP⁺ again once spent.
One such process is the Calvin cycle, the foundation of the light-independent reactions, which uses NADPH and ATP to drive the synthesis of metabolically useful carbohydrates. Plenty more details are seen in the diagram, but this is the only level of detail needed to understand what comes in and what goes out.
In order to perform reduction of NADP⁺, the light-dependent pathways need electrons, because that’s what reduction means.[2] In the most common form of photosynthesis, these electrons are sourced from water, producing hydrogen ions (free protons) and diatomic oxygen in the process. Since carbon dioxide hasn’t even entered the picture yet, we can very confidently say that this excess oxygen is indeed sourced from the water that came into the process, and not carbon dioxide.
But we all know carbon dioxide is an important part of photosynthesis. What’s its role, and where does it go? The short answer is “carbohydrates”. The Calvin cycle performs carbon fixation by adding H₂O and CO₂ to sugars in a convoluted process that ultimately manifests a spare sugar from these ingredients, which is then processed into starch and sucrose that are used in the broader scheme of unrelated metabolic processes. Some of that could be used for respiration, ultimately freeing CO₂ back into the environment, but the main thing a plant does with all that carbon dioxide and water is to make more plant – in fact carbon dioxide and water are where most of the plant comes from.
“Carbon dioxide becomes carbohydrates” isn’t the complete story, however. Carbohydrates have similar amounts of carbon and oxygen atoms, while CO₂ has a readily apparent imbalance. The resolution to this riddle lies in phosphate – as the Calvin cycle churns through 18 ATP molecules to make a single hexose, it leaves behind the same amount of ADP and inorganic phosphate,[3] and every pair of ADP and phosphate has one more oxygen atom compared to the ATP it came from. When CO₂ is fixed alongside H₂O by the action of the enzyme RuBisCo, a pair of glycerates are produced with the expected excess of oxygen compared to carbon. These glycerates are soon made into phosphorylated glyceraldehyde sugars possesing the proper carbohydrate ratio of one oxygen per carbon, with each releasing an inorganic phosphate group in the process. To fix enough carbon for a whole hexose, a total of twelve such sugars will be processed, and twelve phosphate groups freed alongside them. So ultimately out of 1 C, 2 H and 3 O, the extra two oxygen atoms go to phosphate while the rest become carbohydrates.
Later when ADP and inorganic phosphate are recombined to regenerate ATP, the excess oxygen will be returned not as gas but as water again, which could be transpired or guttated or wind up anywhere. This all leaves the possibility that oxygen from CO₂ ends up in PO₄, and then also happens to be returned as H₂O instead of ATP, and just maybe that exact water molecule will then find its way back to photosystem II in the unspecified future, all to finally be freed as one half of diatomic oxygen – but at that point it’s still ultimately come from a molecule of water, and to say that means oxygen comes from carbon dioxide, you might as well cut out all the middlemen and just say it comes from dying stars. Otherwise, oxidation of water in photosystem II remains the most immediate progenitor, particularly as it pertains to plants, and not some side-effect of carbon fixation.